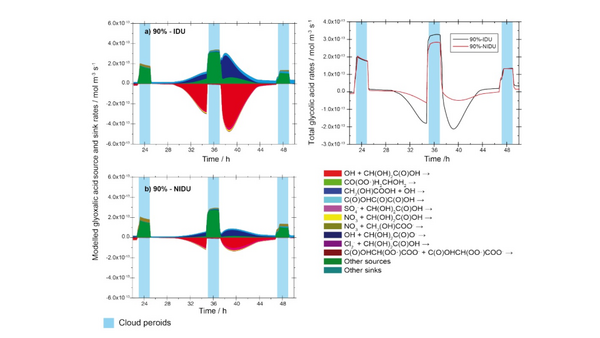
Fig 1. Modelled chemical sink and source mass rates of glyoxylic acid in the aqueous phase for the urban scenario for 90% relative humidity. a) Ideal solutions, b) non-ideal solutions, c) corresponding total rates. [Rusumdar et al., 2020]
Detailed process models that can be used for sensitivity studies as well as for interpretation of laboratory and field data are necessary to understand multiphase processes. A main objective here is the development of aerosol and cloud modules that combine complex multiphase chemistry with a detailed description of microphysical processes, and their application in three-dimensional atmospheric models
The simulations of multiphase chemistry processes consider size-resolution and different particle compositions. Droplet activation, phase transitions between gas and particle phases, and a large number of heterogeneous reactions within the droplet and on the particle surface are explicitly described. These computations require consideration of non-ideal solutions [Rusumdar et al., 2020; Rusumdar et al., 2016]. In addition to the aqueous phase, the focus is increasingly on the organic phase [Gatzsche et al., 2017].
In the research area multiphase chemistry modeling, detailed aqueous phase mechanisms are developed and applied within numerical models studying the physico-chemical multiphase processing in cloud droplets and deliquescent aerosol particles. The main focus of the model applications is given to the analysis of aqueous phase oxidations und their effect on the tropospheric oxidation capacity. For modelling of the complex tropospheric multiphase system, a spectral 0-dimensional box model as well as the detailed air parcel model SPACCIM (SPectral Aerosol Cloud Chemistry Interaction Model; [Wolke et al., 2005]), which were developed within the modelling department, can be used. Currently, the most complex applied multiphase mechanism consists of the gas-phase mechanism MCMv3.2 with more than 13000 reactions and the aqueous-phase mechanism CAPRAM (Chemical Aqueous Phase Radical Mechanism) with 7118 aqueous-phase reactions in the latest version CAPRAM 4.0 [Bräuer et al., 2019]. The phase transfer of currently 276 soluble species is treated in the model after the approach by Schwartz [1986].
Fig 1. Modelled chemical sink and source mass rates of glyoxylic acid in the aqueous phase for the urban scenario for 90% relative humidity. a) Ideal solutions, b) non-ideal solutions, c) corresponding total rates. [Rusumdar et al., 2020]
Bräuer, P., et al. (2019), Development of a protocol for the auto-generation of explicit aqueous-phase oxidation schemes of organic compounds, Atmos. Chem. Phys., 19(14), 9209-9239, doi:doi:10.5194/acp-19-9209-2019.
Gatzsche, K., Y. Iinuma, A. Tilgner, A. Mutzel, T. Berndt, and R. Wolke (2017), Kinetic modeling studies of SOA formation from α-pinene ozonolysis, Atmos. Chem. Phys., 17(21), 13187-13211, doi:doi:10.5194/acp-2017-275.
Rusumdar, A. J., A. Tilgner, R. Wolke, and H. Herrmann (2020), Treatment of non-ideality in the SPACCIM multiphase model - Part 2: Impacts on the multiphase chemical processing in deliquesced aerosol particles, Atmos. Chem. Phys., 20(17), 10351-10377, doi:doi:10.5194/acp-20-10351-2020.
Rusumdar, A. J., R. Wolke, A. Tilgner, and H. Herrmann (2016), Treatment of non-ideality in the SPACCIM multiphase model – Part 1: Model development, Geosci. Model Dev., 9, 247-281, doi:doi:10.5194/gmd-9-247-2016.
Schwartz, S. (1986), Mass-transport considerations pertinent to aqueous phase reactions of gases in liquid-water clouds, in Chemistry of Multiphase Atmospheric Systems. NATO ASI Series (Series G: Ecological Sciences), vol 6., edited by W. Jaeschke, pp. 425-471, Springer, Berlin, doi:10.1007/978-3-642-70627-1_16.
Wolke, R., A. M. Sehili, M. Simmel, O. Knoth, A. Tilgner, and H. Herrmann (2005), SPACCIM: A parcel model with detailed microphysics and complex multiphase chemistry, Atmos. Environ., 39(23-24), 4375-4388.